Introduction to Plastic Materials
Discover the fundamentals of plastic materials, their types, properties, and applications. Learn how plastics have revolutionized industries and everyday life with their versatility and durability. Explore the environmental impact of plastics.
Introduction
Plastics are very versatile materials that we use every day. Understanding plastics materials help us to select the right one for a given application and it also helps to develop improved plastic materials. Unfortunately, plastic training is uncommon and most of the people working with plastics have had no formal training. Furthermore, plastic training is usually in the form of a degree course which means it takes years and involves a great deal of theory.I wanted to help the masses of people who need to understand plastics in a quick and easy way. This page aims to explain plastic materials in the simplest terms possible. Sometimes people ask whether I am “dumbing it down” for them because I have a PhD in plastics and the explanations are so simplistic. They are amazed to learn that I am not making it simple for them and that I actually use these same analogies every day when I am conceptualizing and designing new materials for the Fortune 500. Many years ago, my professor showed me that real experts are able to explain complex topics in a simple way whereas false “experts” hide behind long words and complex theory. So, if you’re ready to understand plastics in ten minutes, let’s get started. It’s much simpler than you expect! First, we explain some concepts in simple terms and then (below) there’s a free 1-hour video with even more information that expands on what you’ve learned.Just Imagine Spaghetti
It really helps to be able to visualize polymer chains but they are too small to see with the naked eye, so it helps to imagine something we know from our everyday lives. I usually think of spaghetti or hair because both are long, thin and flexible like polymer chains are. Thermoplastics like polyethene, polypropylene, PVC, polystyrene and nylon get their strength from polymer chain entanglements. If the chains are too short then tangles cannot form so there is no strength. Imagine very short spaghetti strands or rice. The short rice grains do not hold together, whereas when you try to lift up cooked spaghetti, the whole bunch comes up in a tangled lump. We will hear more about chain entanglements in the upcoming sections. Thermosetting polymers have a different structure. Instead of entanglements, the molecules are all joined together in a network instead of linear chains. The 3D network is strongly bonded and is effectively one giant molecule. Therefore, thermosets do not flow when heated. A common example of a thermoset would be epoxy resin. The two components of an epoxy resin (monomers) are mixed and chemically react in an irreversible way. Many elastomers are thermosets but made of flexible components instead of rigid ones. Another way to visualize thermosets would be to start with the heap of spaghetti and glue the strands together at several points. The glue blobs would represent so-called “cross-links” in the thermoset, i.e. chemical bonds holding the network together and preventing flow.
Polymer Chains are Very Long
Degradation and Stabilizers
Laypeople believe that common polymers do not degrade whereas, in fact, many common polymers do degrade. In particular, polypropylene is so unstable that it cannot be used without adding stabilizers to protect it from oxidation. Similarly, PVC is so unstable that it cannot be melted and processed without stabilizer to protect it. Polyethene is significantly more stable but still oxidizes at room temperature, just at a slower pace than polypropylene. Polystyrene is very stable in the dark but is degraded when exposed to UV light, i.e. in sunlight. What exactly happens when polymers degrade? Well, the chemistry depends on the polymer in question. Many polymers degrade by radical routes. Polyethylene and polypropylene as examples. Other polymers may degrade due to exposure to heat and water. Polyesters and to a lesser extent nylons are prone to that type of chemical attack. For the most part, degradation breaks the polymer chains so they become too short to entangle so strength is lost. In some cases the opposite occurs, i.e. the degradation creates cross-links whereby the chains get ties together more tightly and the polymer becomes brittle. The successful use of plastics depends on the proper selection of antioxidants and stabilizers to protect them. As an example, polypropylene without stabilizer loses it’s strength and fails in about one year at room temperature. Adding a small amount of the correct type of stabilizer can protect it for several decades depending on what conditions it is exposed to.Plasticizers
Plasticizers are oils used to make plastic softer and less brittle. Using our spaghetti or hair analogies, it’s rather easy to visualize how they work. What happens when we put olive oil on our pasta? The stands are lubricated and when the pasta is lifted, the tangles flow apart spontaneously. Likewise, when we put Argan oil in hair it becomes easier to comb. So, it’s now clear why plasticizers decrease the strength of plastics and improve flow. By making it easier for the polymers to move and detangle, the strength is decreased.
Stiffness
The stiffness of plastic is called the modulus. The tensile modulus is measured by pulling the part and measuring the force needed to stretch it by a certain amount. The flexural modulus is where a force is applied to bend the plastic part by a defined amount. The two types of modulus are usually the same or similar because the same property is being measured.
What affects stiffness? Let’s return to our plate of spaghetti. It’s easy to understand that cooked spaghetti, which has floppy strands translates to a low stiffness (low modulus). In the polymer world, soft polymers like silicones, EVA or PE have flexible polymer chains. Other polymers, like Kevlar, have straight, stiff chains like uncooked spaghetti taken right from the package.
Safety
Polymers tend to be safe for several reasons. One is that the molecules are so large that they cannot move around. The polymer chains normally stay where you put them and do not migrate. Any migration is therefore due to small-molecule additives in the polymer because they are mobile. Similarly, pure polymers have no smell because the molecules are too large and involatile to become airborne. Any smell coming from plastics, e.g. new car smell, is due to additives, particularly plasticizers. Some polymers are intrinsically flame retardant but some types like PE and PP burn readily. A wide range of flame retardant additives can be used to ameliorate that issue when it arises. Thus, plastics are safely used in cars, appliances, airplanes and in our homes and have been for decades.
Temperature, Tg and HDT
The effect of temperature on plastics is as one might expect based on common sense. Just like other substances, when plastics are cooled down they become stiffer and more brittle. At low temperatures, the polymer chains have less energy and cannot move around as easily. The chains are therefore stiff and so is the plastic made from them. The temperature at which the plastic changes from flexible to brittle is called the “glass transition temperature”, or Tg for short. Below Tg, the polymer is stiff and brittle like glass.
The glass transition temperature is not a fixed quantity because it changes depending on how you test for it. Think of a strobe light. If you use a strobe light on people dancing, it looks like they are still during each flash, but in reality they are moving. Measurement of Tg works the same way. If you measure Tg using a rapid technique like NMR, it looks like the chains are still, even at quite high temperatures. Using a slower test like DMA would show the chains moving at the same temperature at which they looked still by NMR. Thus, the Tg measured by NMR would be high compared to DMA or DSC. It was discovered that for polymers time and temperature are interchangeable. If you want to learn more about that, then search for “WLF equation”.
Polymers soften as they are heated, i.e. the modulus decreases. The heat distortion temperature (HDT) is a measurement to determine the temperature at which a plastic becomes too soft to support a load. That’s a rather subjective concept as it depends on the load in question so the measurement standards allow for different loads (weights) to be used. The HDT is measured by looking at the bending of a sample as it is heated, so it’s a technique that measures the whole sample. Vicat softening temperature is another way of measuring the softening point and usually gives similarly results to HDT. However, as Vicat is measured by pressing a needle into the part surface, it can give different results to HDT in cases where the part surface has different hardness than the interior of the part.
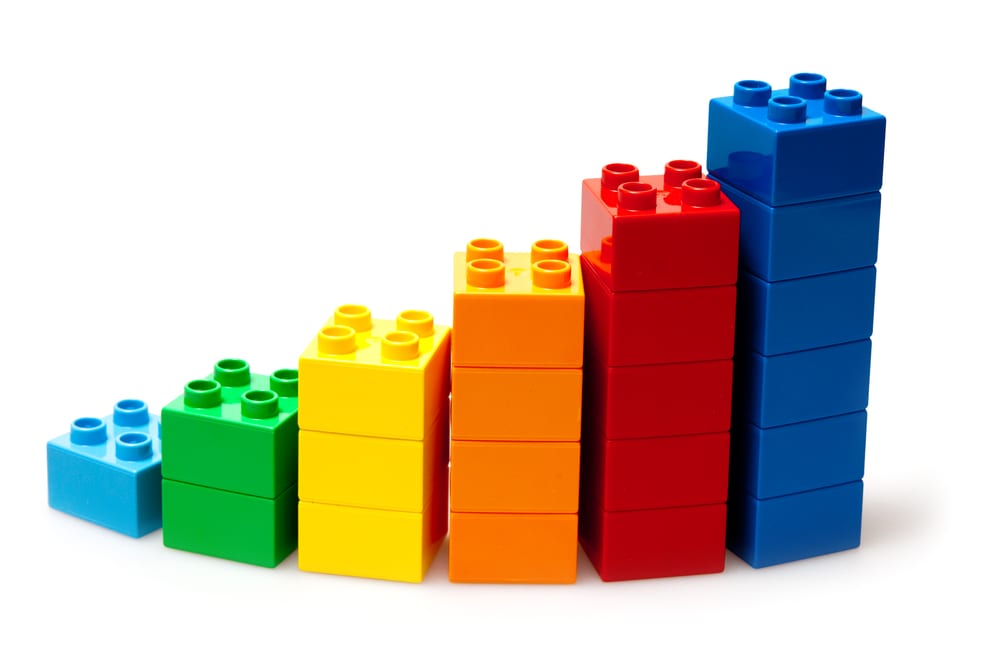
Impact resistance
Some polymers, like polycarbonate, are famous for excellent impact resistance. Others, like polystyrene are hard and brittle so they can easily break when dropped. Compared to the other properties, impact resistance is a little harder to understand and predict. As a rule of thumb, polymers with flexible chains resist impact well. When they are exposed to an impact, the chains simply move and then bounce back, like a rubber ball would. Rubbers (also called elastomers) are a type of plastic with very flexible chains. Polystyrene has stiff chains that cannot deform when dropped so the only way for them to deal with the energy is for the chains to break and the part shatters.
On a side note, you will hear people talking about “impact strength” but that is a misnomer. Impact resistance is the energy needed to break the sample whereas strength is the force needed to break the part. Therefore it is incorrect to say “impact strength” even though almost everyone does.
Flow or Viscosity
It should be apparent that flow can be improved by using shorter polymer chain (lower molecular weight), adding plasticizer or increasing the temperature.
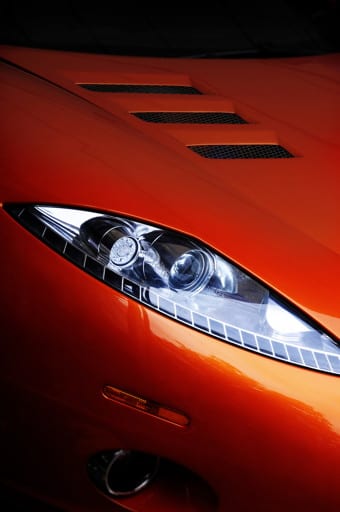
Transparency
In many applications plastics are used for their transparency but not all plastics are transparent. Why is that? Polyethylene is not transparent and polystyrene is. High impact polystyrene is not transparent but white in its natural (unpigmented) form. It turns out that the amorphous (fancy word for non-crystalline) polymers like polystyrene, polycarbonate, SAN and PMMA are transparent. That’s because they are made up of just one phase (type of material). The light passes through them without hitting anything that bends or scatters it.Semi-crystalline plastics like PE, PP and nylons tend to be hazy because they are made up of two phases that interact differently with light.The amorphous (non-crystalline) regions are the continuous phase and inside that are tiny crystals.Usually the crystals have a different refractive index than the amorphous phase, so light is scattered when it travels from an amorphous region through a crystal and then back out again. This results in a white or hazy effect. In fact, any particle with a different refractive index will behave that way. That can include impact modifiers, which make HIPS and ABS milky in colour. It also includes air bubbles, which is why foams are not transparent. The amount of light scattering depends on the amount of second phase, the refractive index difference between the phases and the size of the particles. Surprisingly simple equations exist to predict the amount of scattering.
Plastics and the Environment
Plastics are materials that have changed and improved our lives in innumerable ways. It’s hard to imagine life without them. For all the positives they bring, many people assume that plastics are a “necessary evil”. However, it turns out that plastics do not deserve that reputation at all. Life cycle analysis (LCA) has shown that plastics are actually greener than traditional materials like wood, metal and glass. Similarly, people assume that plastics use a great deal of oil in their production when the facts show the opposite. Although oil is indeed used to make plastics, the net affect of using plastics is to reduce oil consumption. How is that possible? Plastics make automobiles lighter which improves mpg and thereby reduces oil usage. Similarly, plastic insulation is very effective which reduces the amount of oil needed to heat our homes.
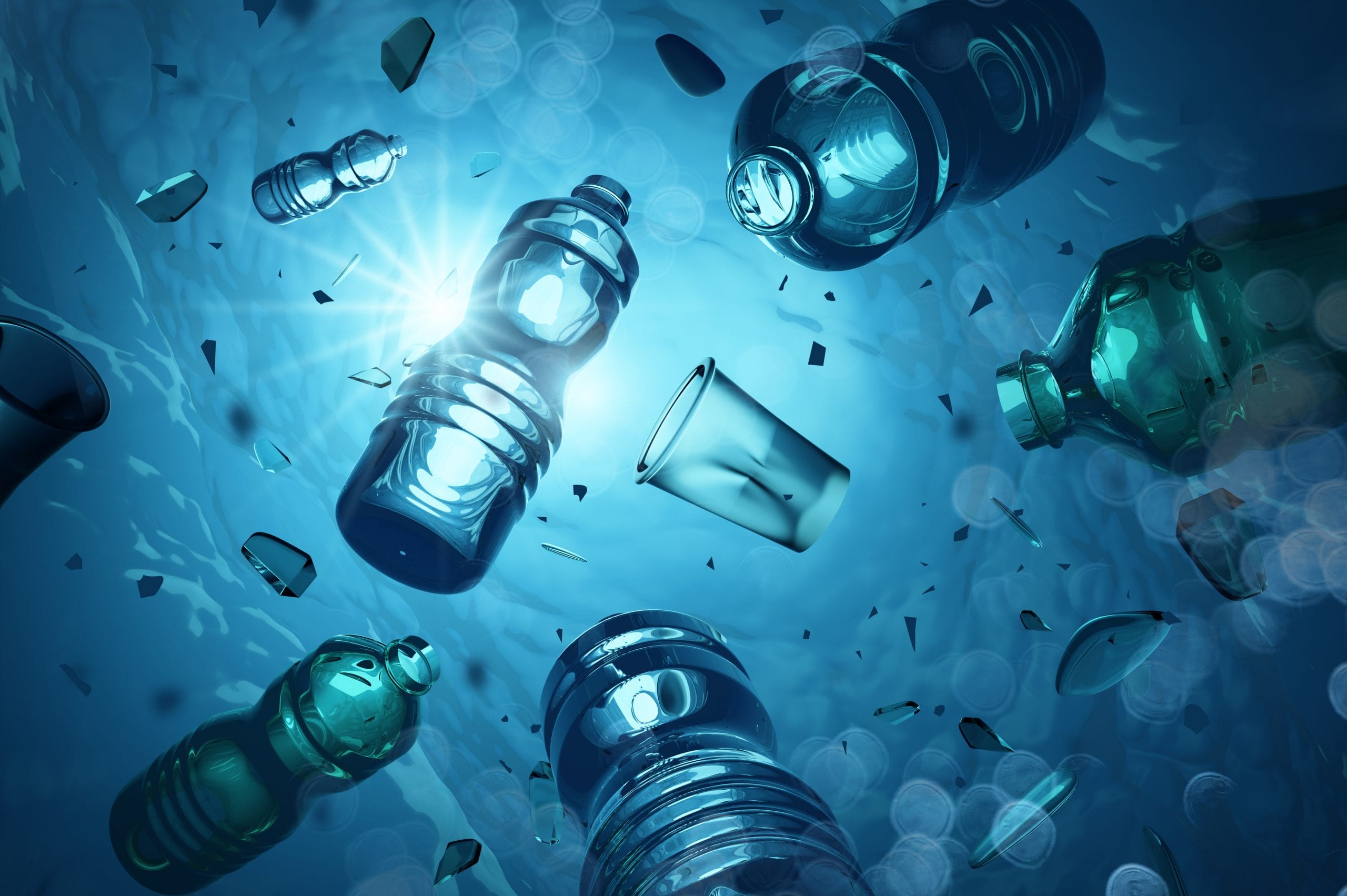
So, although 4% of oil is used to make plastics, the two effects just mentioned combine to result in a reduction of oil usage. Of course, plastics can contribute to litter but that is not the fault of the plastics. Plastics do not throw themselves on the floor or in the ocean. People do that. Anyone blaming plastics for litter just needs to look in the mirror to see whether the real problem is Biodegradable polymers is in vogue at present but it should be remembered that PHB, PHA and PLA have been around for a long time (decades) and have gained very little commercial traction. Such polymers tend to have a worse cost to performance balance and they turn out to be less green that synthetic polymers like PE. I recently read an article saying that PHB would be the next big thing. I had to point out that it was first introduced by ICI in the 1980s and it still has not taken off for the reasons just described.
Want to Learn More?
Learning about plastic materials can be intimidating. Even with the best of intentions, it is hard to teach yourself. There are many good books available but they go into too much detail so you can’t see the wood for the trees. Also, they tend to teach tedious theories with plenty of mathematics thrown in. Well, the good news is that there is a better way. By distilling the basics down into a one hour lecture, it is possible to learn everything you need to get started with plastics. In my career, I have often had the pleasure and challenge of giving people an introduction to plastic materials so that they could get a fast start in their new job. That experience has allowed me to build a set of tools, stories and metaphors that will explain plastics in a simple and fun way. I can promise you there will be no mathematics whatsoever and no theories that make your head spin. You will learn just what you need to know to succeed in your job.
- What are plastic materials?
- What properties do they have and why?
- How do they compare to other materials
- Processing and flow
- Mechanical properties
- Performance : cost so you can find the cheapest plastic for your application
- Amorphous versus semi-crystalline polymers
- Additives
Benefits of the Seminar
- Get a fast start in a new area
- Be more effective in your job
- Gain confidence so you can enjoy your projects more
- Build a solid foundation to build upon
Who Should Attend?
New graduates who are working with plastic materials for the first time or people who have transferred to a job requiring plastics knowledge. Whether you are in R&D, product development, marketing or sales, you will benefit from understanding the materials you work with. R&D people can manage their projects more effectively. Marketing people can better promote products and explain the value proposition. Sales people can be more persuasive by highlighting advantages and through new-found confidence.
Full video available below…